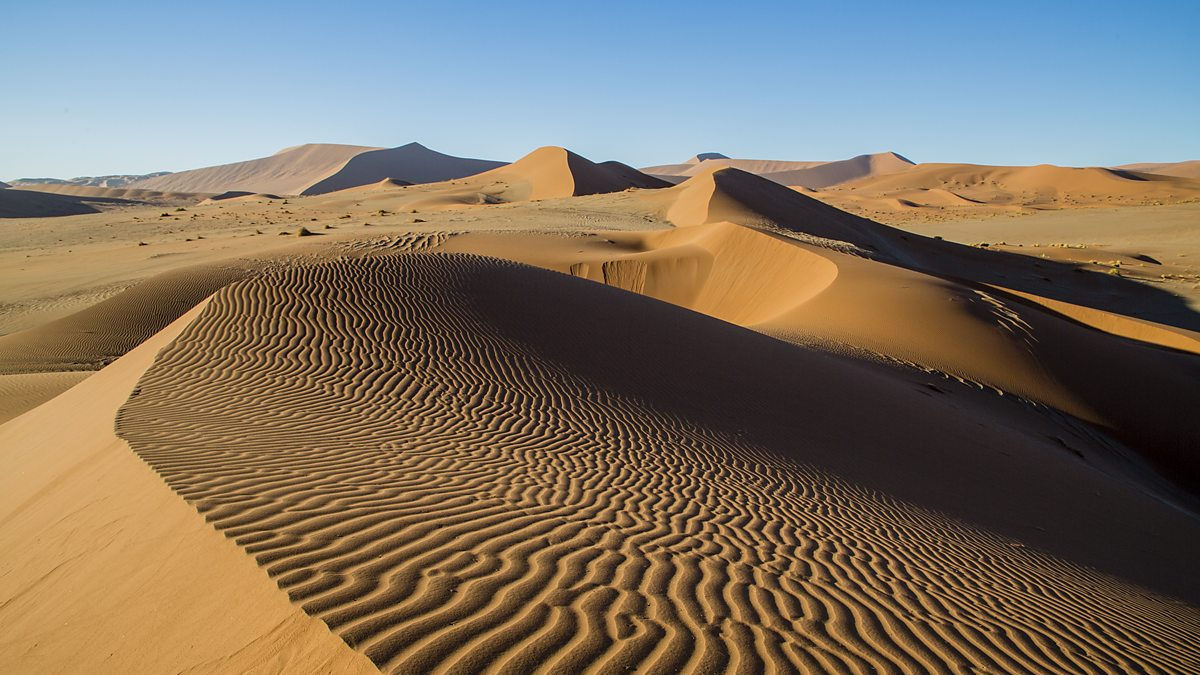
INTRODUCTION
The geophysical methods used by the Group (Geoelectrical, Induced Polarization, Electromagnetic Induction, Georadar, Reflection Seismic) are widely employed for the exploration of natural resources, for applications related to environmental problems and for the study of cultural heritage. These methods have enormous potential due to their capacity to characterize the sub-surface in a precise and noninvasive form, and therefore, have an ample range of applications: hydrogeology, mining, archaeology, geomorphology and environmental contamination studies, among others. They allow characterizing different physical parameters of the materials composing the subsoil (electrical resistivity, polarizability, dielectric permittivity, magnetic permeability, seismic velocity). Since the materials have different responses to different types of excitations, some methods can give very good results whereas others can completely fail, either locally or regionally, for a particular application. Because of this, to increase the resolution and diminish the ambiguity of the solutions, it is usual to apply several prospecting methods together and/or to use methodological variants within a given method, so that the results complement each other. In the last years, the Group has made many innovative advances that allowed remarkably improving the results obtained with these methods in numerous applications. These innovations include the development of new equipment, as well as the introduction of improvements in the techniques of data acquisition, processing and interpretation.
Geoelectrical Method
In the case of the Geoelectrical method, basically, current is injected into the soil through a pair of electrodes, and then the difference of potential generated in a second pair of electrodes is measured, which contains information on the electrical resistivity (or its inverse electrical conductivity) of the subsoil. The reached investigation depth depends on the resistivity of the subsoil and the maximum electrode separation employed. The Group has a multielectrode equipment Saris 500 (Scintrex) for shallow studies (depths up to some meters) and two systems of own development: a multielectrode equipment for superficial and medium depth geoelectrics (30 electrodes, maximum electrode separation 300m) and an equipment for deep prospecting that uses a powerful current source and allows electrode apertures up to 3km. With this last system, depths of several hundreds of meters can be achieved. Usually, data are acquired along lines, and then those data are interpreted by applying 2D inversion methods that provide images of the electrical structure under each measured profile. 3D inversion methods are used only in very precise cases, because to be able to apply them it is necessary to perform a relatively dense grid of measurements in the study area, and this requires a much more prolonged fieldwork.
Georadar Method
GPR systems contain an antenna that emits electromagnetic pulses and a receiving antenna that detects the reflected signals produced when the dielectric permitivity of the subsoil changes. For emitted signals with lower frequency, the obtained penetration is greater but the resolution is lower. The usual methodology consists of covering the study area by moving together the transmitting and receiving antennas, on the surface of the ground, along parallel lines. The Group has a bistatic Georadar PulseEKKO Pro, with antennas of 100, 250, 500 and 1000 MHz. When the acquired signals present low signal-to-noise ratio (for example, in the case of deep targets and/or targets with low contrast with the embedding material), or when the absorption is high, the effectiveness of the method diminishes remarkably. The same occurs when the signals present low continuity, as for example, in very heterogeneous grounds or with irregular surfaces. A way to improve the efficiency of the method in these cases is by using multiple-covering techniques, in which the distance between the emitter and the receiver is varied, and thus more information is acquired on each sounding point. Although some of these techniques are common in seismic, they are rarely used in Georadar. In Argentina, these techniques practically have not been used, with the exception of the works carried out by our group, in which oriented transmission is studied using arrays of emitters (Bullo et al., 2016, 2017, Cedrina et al., 2009, 2011). By using non-usual emitting-receiving configurations and/or by making innovations in the methodologies of data processing and interpretation (p.e. migration 3D), the GPR signals obtained in complex cases, such as the ones mentioned, can be improved.
Electromagnetic Induction Method
Finally, the Group has a multi-frequency SLEM system GEM-2 (Geophex). This portable instrument consists of two coplanar small coils, separated by a fixed distance, and is moved over the area of study at a constant height. One coil generates an inductive magnetic field that oscillates at frequencies between 330 and 47970Hz, and the other detects the secondary magnetic field produced by the currents induced in the ground, which contains information on the electrical conductivity and the magnetic permeability of the subsoil. The achieved penetration depths are typically of the order of tens of meters. As this equipment does not require direct contact with the ground, it allows to survey extensive areas, acquiring great amount of data in relatively brief times. This method is much faster than geoelectrics, and also faster than GPR. Nevertheless, even today, it is still mainly used as a detector of anomalies, through direct visualization of the graphs of the data. To obtain quantitative results, at the most, 1D inversion methods are applied. This relative delay in the development and use of inversion methods, in comparison with the case of geoelectrics, is centrally due to the numerical difficulty that posses the calculation of the response of realistic 2D and 3D structures to magnetic dipoles. The investigation in this subject has great relevance at the moment, and are crucial for taking better advantage of the information contained in the SLEM data.
MAIN CONTRIBUTIONS PRESENTED DURING THE PAST FEW YEARS
Theoretical and Methodological developments
1. Geoelectrical Method
When the equipment Saris 500 was acquired, we began to work with 3D configurations. In order to analyze these data, a 3D inversion code was used, which from a joint analysis with electromagnetic data, allowed to obtain high resolution 3D electrical images of archaeological structures for the first time in the country (Osella et al., 2005).
In addition to the two geoelectrical equipments constructed by the Group mentioned previously, we also developed a geoelectric system with movable platform that allows jointly moving a group of electrodes. This greatly improves the efficiency in field, and lets to cover ampler areas in much less time. Now, we are developing a geoelectrical system with capacitive contacts. As it does not require to bury any electrodes, this equipment reduces the acquisition times even more, and makes possible the use of the method in sites with hard floor, like for example, industrial plants, gas stations and historical constructions. It is necessary to consider that the geoelectrical method is one of the least affected by electromagnetic noise, which makes it especially suitable for measurements in urban or semi-urban areas. At the moment, no such equipment has been developed in Argentina. Though commercial equipments of this type have been developed by a few international companies (for instance, the Ohmmapper by Geometrics), they have a strong limitation (apart from its high cost) as far as the quantitative interpretation of the results is concerned. In these equipments, the interpretation is done by making an analogy with conventional geoelectics (this is, assuming a continuous current source and galvanic contacts), but the equipment actually works with alternating current and capacitive contacts, therefore, this analogy is valid only in a restricted range that depends on the frequency of the emitted signal and on the electrical characteristics of the media. This range of validity is not easily evaluable in commercial equipment, and so, the possibility of quantitatively analyzing the data is wasted.
2. Ground Penetrating Radar (GPR) method
We developed a method for the simulation of GPR fields to investigate soils with appreciable topography (Bonomo y otros, 2004). In general, it is rather infrequent in the studies of the shallowest portions of soil to include the topographic characteristics and their effects. But these effects become important in many cases with abrupt discontinuities or much inclination in the air-soil interface, as usually occur in archaeological prospecting, engineering-structure and geological characterization. In all these cases, it is important to consider the effects of the topography to properly interpret the GPR signals and characterize the subsoil.
We combined direct modeling and migration methodologies to characterize archaeological structures constructed with raw-earth materials (Bonomo y otros, 2009). These structures often produce low-quality reflection signals when they are constructed with very similar materials to those surround them, as usually occurs in many archaeological sites of Argentina and around the World. In these cases, low-permitivity contrasts occur, mainly because of small differences in compaction and clay content (due to addition or sieving during the construction), so it is necessary to adapt and combine different methodologies to detect and interpret the signals of the structures.
We also developed a method based on synthetic transmitter arrays to focus the transmitted field on different structures of interest, thus concentrating the energy on them and reducing the clutter from peripheral zones (Bullo et al., 2017, Cedrina y otros, 2010). This method is useful to resolve reflections that show low lateral continuity and low amplitude with respect to the surrounding noise, as occurs in many studies of strata, fluids, etc.
3. Electromagnetic Induction Method
We developed a code to calculate the electromagnetic response of 2D structures considering the spatial dependence of the source. This method allowed making numerical simulations of data corresponding to geomagnetic deep soundings (GDS) and, from this, obtaining estimations of the sensitivity and resolution of the GDS for the characterization of certain structures (Martinelli and Osella, 2003).
In addition, we developed a method for calculating the response of 2D structures to magnetic dipoles. Given the acquisition of the equipment GEM-2, the aim was to obtain a method for estimating the response of different kind of structures to this type of sources. The developed method turned out to be very effective for the characterization of various buried structures and for the study of contamination plumes (Martinelli et al., 2006; Lascano et al., 2006).
As it was indicated previously, to model the data collected with SLEM equipment like the GEM-2, 1D inversion methods are applied. Then, combining those results, 3D images of the subsoil are constructed. One of the factors that strongly deteriorates the quality of these images is the fact that the data are usually affected by cultural noise. Considering this, methodologies of data acquisition and filtering were developed that enabled to obtain 3D images with much better resolution, even in urban zones, where the signal-to-noise ratio is particularly poor (Martinelli and Duplaá, 2008; Osella and Martinelli 2010).
4. Models of diffusion phenomena in anisotropic media
We have studied diffusive phenomena in anisotropic media with the initial goal of applying the results to fluids in porous media. However, from the interaction with researchers in anthropology held at the same time, these investigations led to the study of the spatial distribution of biological organisms, particularly for a first application to the settlement of anisotropic environments. This behavior can be described as a diffusive phenomenon plus a term that takes into account population growth. One of the most used models in population dynamics is the model proposed by Fisher and studied almost simultaneously by Kolmogorov, Petrovsky and Piskunov (F-KPP equation). In this context, we developed a numerical solution to the equation F-KKP in anisotropic media and then performed simulations in order to compare results from evolution considering deterministic and stochastic behaviors, which were applied to the modeling of the initial human dispersion in America (Martino et al, 2007; Lanata et al 2008).
Applications
The described developments in the prospecting methods (referred to the measurement instruments and the techniques for data acquisition and interpretation) were introduced due to the necessity of solving specific problems, concerning different concrete applications for which the only way to obtain an adequate characterization of the structures and/or to be able to explain the detected phenomena, was to introduce improvements to the conventional uses of the methods. Some of those applications are the following:
1. Characterization of hydrocarbon contamination plumes
The first works in this line were performed using scale models to determine the potential of the geoelectric method from 2D profiles. The possibility of applying these developments to real situations arose when Repsol-YPF asked us for advice for characterizing sites contaminated by spills from the drain pools located along an oil pipeline in Las Heras, Province of Santa Cruz, Argentina. The goal was to find the best methodologies to characterize the contaminant plumes, because considering their locations from borehole data, and the thicknesses of the supernatant layers, in order to obtain successful results, the techniques usually employed should be modified. We studied about ten areas, each one with different characteristics, which gave us an interesting insight about how to deal with this kind of studies. The first two works completed were published as case histories (Osella, and others, 2002 and Vega et al, 2003).
A line that emerged from these studies was to analyze the age of the spills through its electrical characteristics. Hydrocarbons often undergo biodegradation processes that produce changes in the electrical properties of the medium. An interesting result was obtained from analyzing the studied cases (Osella et al, 2003). We found that contrary to what happens when the oil is housed in sandy environments, when it is contained or is in contact with clays, the biodegradation process is slowed. This result was one of the first to be reported and is often cited (see eg, Che-Alota and others, 2009).
Then, this line was continued by studying hydrocarbon spills in urban and semi-urban areas. Through a collaboration project with Universidad Abierta Interamericana, we studied several spills and designed pos-remediation monitoring strategies (Coria y otros, 2009). In addition, from the study of an accident in an oil duct at an urban zone, we developed a strategy to estimate the extent of the contamination in the soil based on the complimentary use of the Geoelectric and SLEM methods, combined with 3D numerical simulations for estimating the distortions introduced in the results by the uncovering of a stretch of the pipe (Martinelli y otros, 2011).
2. Corrosion effects due to induced currents in buried pipes – Pipe characterization
We investigated the effect of the soil resistivity on currents induced in buried pipes and the effects of corrosion. The works in this line provided an answer to a concrete problem that could not be solved by the usual methods. In certain zones along a pipeline, parasitic currents appeared that could not be compensated by the cathodic protection systems. It was suspected that origin of these currents was associated to geomagnetic activity, but it was unclear why they only appeared in certain particular zones. To analyze this problem, we developed a model to simulate the induced currents assuming the natural field as the induction source. We found that the induced currents followed the behavior of the external field and, most importantly, they increased when the pipeline crossed resistive zones. This result is totally novel, since the usual practice is to increase the cathodic protection when a pipe crosses conductive areas, as this areas are considered much dangerous from the point of view of corrosion risk. Tests were performed throughout pipeline systems located in Tierra del Fuego and Buenos Aires (Osella and Favetto, 2000; Osella et al., 1999, 2002). In order to quantify this effect, controlled experiences were made in our laboratory, from which the dependency of the corrosion rate with the resistivity of the ground was corroborated. Increments of almost 40% were found in resistive grounds for inductive fields with frequencies of the order of 50 Hertz (Osella et al., 2003; Lopez et al., 2006).
We also developed GPR methodology to detect pipe flanges by measuring from the ground surface, without excavating or stopping the normal operation of the pipeline and applied it to different case studies (Bonomo et al., 2011). Likewise, we developed methodology to determine with precision the orientation of pipes in places that do not allow good access or mobility on the surface, such as narrow paths, corridors, etc. (Bullo et al., 2016).
3. Archeology and preservation of cultural heritage
The group has been pioneering in our country respect to the application of geophysical methods for the characterization of archaeological remains prior to excavations. These studies offer results of inestimable value for the archaeologists, since they allow them to save great amount of time by precisely determining which areas to excavate and/or protect. Different methods were proposed, and methodologies of analysis and joint interpretation of geophysical data were developed in order to obtain high resolution maps, adapted to the characteristics of the sites and environments, as well as to the archaeological objectives on each case.
The first studied case came through an interdisciplinary collaboration project with the group of Historical Archaeology of the School of Philosophy and Arts, for the characterization of the archaeological site Floridablanca, in San Julian, Province of Santa Cruz, Argentina. The results obtained from the combination of electromagnetic data (Georadar and SLEM) and 2D and 3D geoelectrical imaging allowed to construct a map of a sector of the site that successfully guided the excavation tasks, which were carried out from these results. This constituted a totally novel project, since at the moment, it was not usual to apply these techniques simultaneously, and in fact in our country there were no previous references of this type of works (e.g., Lascano et al., 2003; Osella et al., 2005).
We have been working in the Fiambalá valley area, a semi-desert region of the Province of Catamarca. Here the environment is very different from the previous one, which implies a modification of the methodologies in order to adapt them to a sandy and dry environment. These studies and results, both geophysical and archaeological, have been very important and of wide repercussion. These have included the location and characterization of various pre-Inca, Inca and Hispanic constructions (Bonomo et al., 2012, 2013, Ratto et al., 2013).
From an agreement with an international organization (World Monuments Funds), we worked in the Jesuitic Mission of San Ignacio, Province of Misiones, to make a study for finding and characterizing unknown buried remains, with the purpose of guaranteeing its preservation (Bongiovanni et al., 2008). Recently, we have been working in collaboration with other group of archaeologists in the Province of Santa Fé. Through this collaboration, it has been possible to find and characterize the remains of the Sancti Spiritus Spanish fort (Bonomo et al., 2012).
We have also carried out archeo-geophysical studies in the Manzana de Las Luces (Bonomo et al., 2016, Osella et al., 2015), where a Jesuit Mission functioned and, later, the Facultad de Ciencias Exactas of the UBA, and in the former Taylor (Osella et al., 2009), where nowadays is the museum of Casa de Gobierno de La Nación. Other studies have been related to cultural structures with characteristics different from the previous ones, such as a monument (Bonomo et al., 2015). In this case we have investigated the internal structure of the pedestal, which provided valuable information for optimal preservation during disassembly and maintenance.
4. Geophysical and environmental studies at Llancanelo Lagoon, Payenia Region, Mendoza Province, Argentina.
The overall objective of this project is to conduct a comprehensive geological-geophysical-environmental research of the Llancanelo Lagoon area and its surroundings, including the a preliminary analysis of the environmental aspects of the aquatic environment, vegetation and recent environmental evolution of the lagoon. One novel aspect is the implementation of terrestrial geophysical methods as a tool for the study of lacustrine media. Several of the proposed studies will be performed for the first time in the region. At present, we have performed a geophysical survey of the subsoil up to a depth of a few hundred meters, in order to recognize the spatial distribution of the stratigraphic sequences, using electrical and electromagnetic methods (Osella and others, 2007; Violante and others, 2008, 2009).
5. Sedimentology
Recently, we have been working on the characterization of different types of aeolian and fluvial deposits, in the provinces of Buenos Aires, La Rioja and Catamarca, to determine their structure and sedimentation dynamics. This is relevant in relation of the construction of geological models, the use of cultivation areas, the evaluation of desertification processes and the protection of inhabited areas.